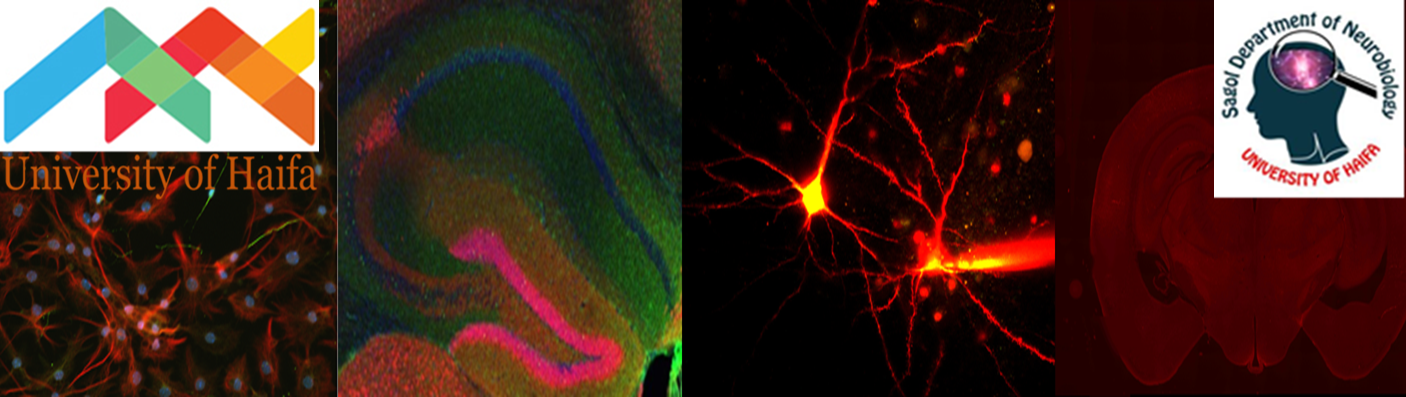
Hanoch Kaphzan lab
Laboratory For Neurobiology of Psychiatric Disorders
Basic Research
Angelman syndrome
Angelman syndrome (AS) is a human neuropsychiatric disorder associated with symptoms that include autism, mental retardation, motor abnormalities, and epilepsy. In most cases, AS is caused by the deletion of small portions on the maternal copy of chromosome 15, which includes the UBE3A gene. This phenomenon, that only the maternal copy will be expressed, is called “imprinting”, and in AS it occurs only in the brain. The UBE3A gene encodes an enzyme termed ubiquitin ligase E3A (also termed E6-AP), which is one of a family of enzymes that covalently attaches polyubiquitin chains to proteins, to signal for their degradation by the 26S proteasome. This molecule is also implicated in schizophrenia. A mouse model of AS with the exact same deletion has been generated and these mice exhibit susceptibility to epilepsy, impaired motor functioning, and cognitive abnormalities that correlate with neurological alterations observed in humans. Like in other cases of autism, one of the main brain regions that have been proven to be affected in human AS and in the AS model mice is the hippocampus. On the molecular level, it was demonstrated that calcium signaling in AS mice hippocampus is impaired, and that this impairment is related to the behavioral impairment observed in these mice. We recently demonstrated that hippocampal CA1 pyramidal neurons in AS mice have increased expression of the a1 subunit of Na/K ATPase (a1-NaKA). It is well established that intracellular calcium dynamics and intracellular calcium concentrations are regulated and even largely determined by Na/K-ATPase (i.e. ↑Na gradient = ↓intracellular calcium). Therefore we are investigating the calcium dynamics of hippocampal CA1 neurons of AS mice and the effect of increased a1-NaKA expression on these calcium signaling pathways. We also examine whether lowering a1-NaKA expression can alleviate the hippocampal impairments in these mice.
Na/K-ATPase (NaKA) untraditional roles in neuronal functioning
Our recent findings show that different NaKA subtypes have distinct functions and display different effects in distinct brain regions. Our aim is to reveal these differences, and to establish the functional role of each NaKA subtype in neural plasticity processes.
Axon initial segment regulation
Axon initial segment (AIS) is the most proximal region of the axon. Under normal conditions the AIS is the cellular site where action potential is triggered, thus functioning as a major signal processing and computation unit of the neuron. This is because it has a unique morphology and composition; Voltage-dependent sodium channels are concentrated and anchored to the AIS membrane, which makes this cellular region with the lowest threshold for triggering action potential. Until recently, the AIS was considered to be a rigid structure with no apparent plasticity. However, new studies have demonstrated that the AIS is modified in response to cellular excitability changes, in the opposite direction, in order to preserve the overall cellular excitability. Nevertheless, not much is known about the underlying mechanisms of these AIS plasticity processes. We showed that AIS of AS mice is altered, and that this alteration is developmental. Our results with the AS mouse model supplied us with a platform for investigating AIS plasticity in-vivo in mammals. We aim to employ this platform and investigate the mechanisms underlying AIS plasticity by employing various manipulations that will alter excitability and signaling pathways and examine the resulting effects on the AIS structure and function.
Trans-cranial Direct Current Stimulation
Trans-cranial direct current stimulation is a novel therapeutic technique used for various clinical applications such as chronic pain, fibromyalgia, epilepsy, Parkinson's disease and major depression. The rule of thumb was that the neurons under the anodic electrode are activated, while the neurons under the cathodic electrode are inhibited. However, we know that this is not exactly the case, and the underlying mechanisms by which tDCS work are yet unknown. DC currents impact neurons depending on their morphology and orientation to the direction of field and the various cellular compartments are effected differently. We do not know what are the molecular events that take place when neurons are being depolarized, and how do these molecular events relate to the observed plastic changes. Even less is known about the chronic effects of DC fields application on the molecular and cellular levels. Therefore, we are investigating the acute (in slices) and chronic (in-vivo) effects of DC fields on neurons from different brain regions, and exploring molecular and physiological effects of these manipulations.



Experimental recording set-up. (a) Custom made experimental set-up with Ag-AgCl electrodes producing uniform electric fields across the slice. Soma and bleb patch electrodes stimulate and/or record the electrical responses. The reference microelectrode used to measure the bath polarization. (b, left) For parallel field stimulation, the electrodes were placed across the slice such that the electric field was parallel to the dendro-axonic axis of the layer-V pyramidal cells, whereas for the orthogonal orientation the electric field is perpendicular to the dendro-axonic axis. (b, right) The axonal alignment, θ, relative to the electric field is determined for each cell. (c) Sample image of a layer-V pyramidal cell, filled with Alexa-488. (red arrow: axon hillock, blue arrow: axon initial segment, yellow arrow: axon bleb). (d) Cellular orientation in the slice is illustrated in the schematic. The axon bleb is patched at the surface of the slice whereas the dendrite remains intact deep inside the slice.